Bioleaching has been found to be widely used in the mining industry because bioleaching is environmentally friendly, saves processing costs due to low energy consumption and low capital investment, and can extract low grade and high grade ore. However, the commercialization of microbial leaching of laterite nickel ore has not yet been developed. The laterite nickel ore reserves account for 2/3 of the offshore nickel resources. Nickel concentrates cannot be obtained by conventional beneficiation methods. Currently conventional method for recovering nickel from laterite nickel ore is pyro metallurgical and hydrometallurgical.
Unlike heterotrophic organisms, chemically-inorganic autotrophic microorganisms take up carbon from carbon dioxide to sustain survival and growth. To treat such carbon sources, they harvest energy by oxidizing inorganic compounds. By oxidizing the compound, the energy stored in the chemical bond is utilized during cell processing. Bioleaching process can be used of the inorganic sulfur and bioavailability autotrophic sulphide minerals (e.g. pyrite) as an energy source, which ultimately generate sulfuric acid (formula (1) to (4)) in the oxidation process.
(1)
(2)
(3)
(4)
The pH of the resulting sulfuric acid retention system is maintained at a level suitable for bacterial growth and contributes to efficient leaching of laterite nickel ore. Hydrogen ions can replace metal ions in the mineral matrix to achieve metal dissolution.
The proton etching process is:
(5)
However, nickel in the laterite nickel ore has not been recovered by chemically-inorganic autotrophic bacteria, which may be due to the lack of sulfur in the ore and thus the desired sulfuric acid. In order to successfully utilize such bacteria, it is necessary to produce sulfuric acid by externally adding sulfur.
Therefore, the purpose of this study was to determine the possibility of using leaching inorganic autotrophic microorganisms to leach laterite nickel ore. In particular, several factors affecting the leaching of laterite nickel ore by chemically-inorganic autotrophic microorganisms are examined. Use a 2-level 5-factor statistical test design method (
The factors affecting bioleaching are examined: particle size, slurry concentration, pH, matrix type (pyrite and sulfur), and bacterial inoculum size.
I. Materials and methods
(1) Mineral samples and characteristics
The laterite nickel ore samples used in this experiment were supplied by the Mintek Institute of South Africa. Use a standard set of sieves to divide minerals into three different grades (-38
m, +53-63
m and +100-150
m). Prior to bioleaching, chemical analysis and particle size composition analysis were performed for each fraction. After the acidic leaching of minerals using aqua regia (a mixture of 3 :1 HCl and HNO 3 ), the chemical composition of the laterite nickel ore was analyzed by atomic absorption spectroscopy. Table 1a shows the abundance of minerals of different components in the laterite nickel ore sample. Table 1b is the chemical composition expressed as oxide in laterite nickel ore. Table 1c shows the particle size distribution of different particle sizes and the elemental composition corresponding to each particle size. In Table 1c, the average particle diameter was calculated from the volume fraction of the particles.
Table 1a Relative abundance of different minerals in laterite nickel ore
Phase | Specific value |
Nickel serpentine [(Mg) 3 Si 2 O 5 (OH) 4 ] | less |
Goethite and limonite [(Fe,Ni)O(OH) and Fe 2 O 3 ·H 2 O] | many |
Pyrite titanium [(Fe, Ti) S 2 ] | Trace amount |
Nickel pyrite [(Fe,Ni)S 2 ] | Trace amount |
Talc [(Mg) 6 Si 8 O 20 (OH) 4 ] | Trace amount |
Saponite [(Ca 0.25 (Mg,Fe) 3 (Si,Al) 4 O 10 (OH) 2 ·nH 2 O)] | Trace amount |
Tremolite [(Ca 2 Mg 5 )Si 8 O 22 (OH) 2 ] | Trace amount |
Quartz [SiO 2 ] | a lot of |
Table 1b Chemical composition of laterite nickel ore samples
Mineral | SiO 2 | Fe 2 O 3 | Cr 2 O 3 | Al 2 O 3 | MgO | NiO | CoO |
content/% | 52.8 | 21.9 | 1.0 | 2.5 | 7.5 | 1.9 | 0.3 |
Mineral | CaO | MnO | CuO | C | S | P | |
content/% | 0.7 | 1.3 | <0.1 | 0.1 | <0.1 | 0.1 | |
Table 1c Elemental composition and average particle size of different grades of laterite nickel ore
Size / m | <38 | 53-63 | 100-150 |
Ni content /% | 1.7 | 1.5 | 1.4 |
Co content /% | 0.1 | 0.2 | 0.2 |
Average granularity / m | 10.4 | 59.5 | 137.1 |
(two) bacteria
The mixed cultures of chemically active autotrophic bacteria (Acidithiobacillus ferrooxidans, Acidithiobacillus caldus and Leprospirillum ferrooxidans) used in this experiment were provided by the Mintek Institute of South Africa. The bacteria were cultured in 9K medium.
(3) Experimental design
In this study, the statistical test design method (DOE) was used to study the leachability of laterite nickel ore. Several process factors were also studied. Several factors are systematically changed to ensure the primary factors studied and the reliability and independence of the interactions between them. The effects of major factors on the bioleaching of laterite nickel ore were studied during the characteristic study phase. The purpose is to identify important variables that affect the results of the leaching and their interactions. Statistical experiment design for each factor and the significant application of factors between them
Method to evaluate. The main objective of the study was to maximize the leaching rate of the chemically-inorganic autotrophic microorganisms to leach the laterite nickel ore. Therefore, the recovery rate of nickel (ie, conversion rate) is the target (response value).
1. Test factors and levels
Although previous researchers used heterotrophic microbes to identify some important factors that affect the dissolution of laterite nickel ore. This test determines the effects of some factors on the leaching of laterite nickel ore by chemically-inorganic autotrophic microorganisms and quantifies them so that these factors can be measured. In this study, potential design factors were divided into control factors and constant factors. The control factors were the factors actually selected in the study (Table 2). The invariant factors are those that may work for the response but are not critical to the test, so they are set at a specific level. (See (4) Normal probability relationship curve of influence)
Table 2 Levels of test factors and control factors
Controlling factor | Level 1 | Center point | Level 2 |
pH | 1.5 | 2.0 | 2.5 |
granularity/ | -38 | -63+53 | -150+100 |
Pulp concentration /% | 5 | 10 | 15 |
Substrate type a | 5% sulfur and Fe 2 + | 5% sulfur and Fe 2 + | 5% pyrite and Fe 2 + |
Inoculum size /% | 5 | 10 | 15 |
The concentration of
a divalent iron ion (Fe
2 + ) is the same as in the literature (Silveman and Lundgren, 1959).
To simplify the calculation, the factors are studied with their encoded values. The physical level and coding level of the factors are shown in Tables 3 and 4. In general, the coded value of a continuous variable is obtained by the following formula: coded value = [actual value - 1/2 (highest value + lowest value)] 1/2 (highest value - lowest value). For qualitative variables, the encoded values ​​are randomly assigned.
In the basic design, the matrix type column is obtained by combining the particle size and the slurry concentration, and the inoculum is obtained by combining pH, particle size and slurry concentration.
Table 3 by
Nickel recovery results from factor test design (basic design)
standard order | immediately order | Control factor a | Ni average recovery rate /% |
A | B | C | D | E |
1 | 3 | -1 | -1 | -1 | +1 | -1 | twenty four |
2 | 8 | +1 | -1 | -1 | +1 | +1 | 20 |
3 | 7 | -1 | +1 | -1 | -1 | +1 | 30 |
4 | 6 | +1 | +1 | -1 | -1 | -1 | 26 |
5 | 1 | -1 | -1 | +1 | -1 | +1 | 17 |
6 | 4 | +1 | -1 | +1 | -1 | -1 | 14 |
7 | 5 | -1 | +1 | +1 | +1 | -1 | 30 |
8 | 2 | +1 | +1 | +1 | +1 | +1 | 26 |
a horizontal table to experimental factors represented by -1 and +1 coded as follows: for the pH (A): 1.5 (-1 ) and 2.5 (1); particle size (B): - 38

m(-1) and -150+100

m(+1); pulp concentration (C): 5% (-1) and 15% (+1); matrix type (D): sulfur (-1) and pyrite (+1); inoculum (E): 5 %(-1) and 15%(+1).
Table 4 by
Nickel recovery results from factor test design (folding design)
standard order | immediately order | Control factor a | Ni average recovery rate /% |
A | B | C | D | E |
1 | 6 | +1 | +1 | +1 | -1 | +1 | 26 |
2 | 1 | -1 | +1 | +1 | -1 | -1 | 29 |
3 | 8 | +1 | -1 | +1 | +1 | -1 | 16 |
4 | 4 | -1 | -1 | +1 | +1 | +1 | 20 |
5 | 3 | +1 | +1 | -1 | +1 | -1 | 32 |
6 | 2 | -1 | +1 | -1 | +1 | +1 | 35 |
7 | 5 | +1 | -1 | -1 | -1 | +1 | 15 |
8 | 7 | -1 | -1 | -1 | -1 | -1 | twenty three |
a horizontal table to the actual coding of -1 and +1 factors expressed as follows: for the pH (A): 1.5 (-1 ) and 2.5 (1); particle size (B): - 38

m(-1) and -150+100

m(+1); pulp concentration (C): 5% (-1) and 15% (+1); matrix type (D): sulfur (-1) and pyrite (+1); inoculum (E): 5 %(-1) and 15%(+1).
2, statistical analysis
The test results were statistically analyzed by applying the influence probability curve, residual analysis and midpoint analysis.
(4) Normal probability relationship curve of influence
When analyzing unresolved factor data, occasional high-order interactions occur, so a normal probability relationship curve is used to evaluate significant factors. This is the cumulative normal probability curve affected by the actual value. Negligible effects are generally normal distribution, mean variance (
2 ) is 0, which is a straight line equation, and the mean variance of the significant influence is not zero and is not a straight line equation.
The response values ​​for each factor level are averaged to get the impact of the statistical design. The difference in the mean of the responses at the two levels of each factor represents the significance of that factor's effect on the response. A mathematical formula indicates that a single effect caused by a change in an input variable can be calculated by the following formula:
(6)
In the large probability relationship curve, all effects need to be graded from low to high and numbered in this order. Next, the effect of the number (i) is obtained by the following formula:
Value =
×100% (7)
(5) Mapping analysis of residuals
The normal relationship curve of the residuals provides a diagnostic test for uncertain, questionable models. The normal probability relationship curve of the data residuals tests whether the assumption that the residual is normally distributed is correct. If the residual is a normal distribution, it is a straight line. The residual fit curve (fitting model) verifies the assumption that the variables have the same bias value in each group. Since outliers may have an undue influence, when the experiment is excluded from the analysis, the residual to be deleted for each experiment is calculated based on the predicted value. The residual is calculated by the following formula:
Residual =
(8)
(6) Using the midpoint to detect curvature
Adding a midpoint to the design protects the curvature produced by the secondary effect and allows independent evaluation of the error polarity obtained. in case
Is the average value of nickel at the nth c midpoint transformation,
Is the average value of the nickel conversion rate of the factor being studied, if
-
Smaller, then the midpoint is located near or across the plane of the factor points, there is no quadratic curve. If
-
If it is large, there is a quadratic curve.
(7) Testing and sampling
all
The bacterial leaching test of the factor design was performed in a 500 mL Erlenmeyer flask. Add 200 mL of sterilized medium, appropriate amount of sterilizing energy, pre-measured bacterial inoculum and a suitable amount of laterite nickel ore to the leaching system and place in a rotating shaker at 30 ° C and 190 r / min. Under cultivation. The pH of the system was maintained using 1 mol/L sulfuric acid and 1 mol/L sodium hydroxide. The experimental system was monitored every two days and pipetted with a pipette (1.5 mL) and then quickly filtered. The reduction in liquid extraction is compensated by the addition of distilled water (or 9K broth). The nickel content in the filtrate was analyzed by atomic absorption. All tests were performed at random until the nickel recovery remained constant.
Second, the results and discussion
(1) Main factors
The data in Tables 3 and 4 are used to assess the impact of the main factors and their interactions (as shown in Figure 1). The nickel recovery after bacterial leaching of laterite nickel in Tables 3 and 4 was calculated based on the percentage of nickel in the liquid phase as a percentage of the total nickel in the laterite nickel ore.
A mixed mode with effects when applying a fractional factor design. This means, (assuming that the interaction between the three factors and the higher factors is not significant), the evaluation effect of one factor is the combination of the actual value effect of one factor and the interaction effect of two factors. In order to distinguish the effects of the main factors from the interaction effects of the two factors that are not statistically significant, a folding technique is used in which all control factors are the original opposite symbol values.
Table 4 shows the opposite values ​​of the basic design on the symbol, that is, folding. Combine these two designs to evaluate all the main effects and remove the interaction effects of the two factors that are not statistically significant, as shown in Figure 1.

Figure 1 Normal diagram of the interaction of major factors and factors
(combined from basic design and folding design); A, B, C, D and E are the main factors
(Table 3); AB, AC, AD, AE, BC, BD, BE,
CD and CE are factors in the interaction
The significant effect is determined by the probability plot shown in Figure 1. In Figure 1, when the basic design and folding design were applied simultaneously to evaluate the effects of individual factors, A (pH), B (particle size), C (slurry concentration) and D (matrix type) were statistically significant factors. Because their mean is not zero. E (inoculation amount) is not a statistically significant factor because it does not differ much from a normal distribution (zero mean). There is an important interaction between the concentration of the slurry and the type of matrix.
When only the main factors of the basic design and the interaction of the two factors are drawn, the main effect cannot be distinguished from the interaction of the two factors in the mixed mode (the result is not shown).
A polynomial model (predictive or fitted model) between significant factors and responses is established to illustrate the relationship between response and significant factors:
R=24.03-2.08A a +5.40B b -1.69C c +1.36D d +0.71C c D d (9)
The negative sign of some of the variables in the predictive model indicates that the recovery of nickel from the leaching of laterite nickel ore is greatest, and these factors need to be kept at a low level. A positive sign indicates that the factor needs to be kept at a high level.
The four recoveries observed at the midpoint were 20%, 21%, 25%, and 22% (Table 5). The average of these four midpoints is 22%. The average of the eight basic designs (Table 3) and the eight folded designs (Table 4) was 24%. Although the two averages are not close (the difference is 2%), there is a possibility of curvature. However, the nonlinear test does not find which factor contains curvature and may not exist. However, for the purpose of this study (factor screening), it is assumed that there is a linearity of approximation. In fact, because equation (9) contains interacting terms, the model can represent the curvature in the response formula.
Table 5 by
Nickel recovery rate result from fractional factor design (midpoint)
Numbering | Control factor a | Ni average recovery rate /% |
A | B | C | D | E |
1 | 0 | 0 | 0 | 0 | 0 | 20 |
2 | 0 | 0 | 0 | 0 | 0 | twenty one |
3 | 0 | 0 | 0 | 0 | 0 | 25 |
4 | 0 | 0 | 0 | 0 | 0 | twenty two |
level 0 indicates practical factors encoded in
a table as follows: for the pH (A): 2.0; particle diameter (B): - 63 + 53

m; pulp concentration (C): 10% w/v; matrix type (D): sulfur; inoculum (E): 10% v/v.
Figure 2 shows the normal probability curve of the residual. As can be seen from the figure, all the residuals are on a straight line, and the linear correlation coefficient is 0.97, which indicates that the residuals are normally distributed. By plotting the residuals to the fitted values ​​(fitting model values ​​or predicted recoveries), it is possible to test the assumption that the deviations for each group are the same (Fig. 3), that is, the distribution of random errors with zero mean and deviations being constant values. All residuals are distributed between -2 and +1 and have no system structure. Since the residual is a constant value, a zero mean, and an independent normal distribution (Figs. 2 and 3), it can be seen that equation (9) can better fit the test data. In other words, the assumption of error is satisfactory.

Figure 2 residual normal distribution curve

Figure 3 Curve between residual and expected recovery
(2) The impact of factors on recovery rate
1, the impact of pH
The effect of pH is shown in Figure 4. As can be seen from the figure, the recovery of nickel is high at low pH. The pH of the medium significantly affects the growth and activity of eosinophils. Since the activity of the bacteria is high at a low pH, it is desirable that the acid concentration (H + ion) is high (pH = -log [H + ]). It is expected that H + ions can cause dissolution of nickel as shown in the formula (4).

Figure 4 Effect of pH on nickel recovery (combined from basic design and folding design)
2, the impact of granularity
The effect of particle size is shown in Figure 5. As can be seen from the figure, within the range studied, below 38
The m particles have a negative impact on the nickel recovery. This is inconsistent with the expectation that more exposed surfaces lead to enhanced nickel dissolution. This is also inconsistent with the fact that the nickel content of the small particles is larger than that of the large particles (see Table 1c). This may be due to the increased collision rate between laterite nickel ore particles and between mineral particles and bacteria as the particle size is reduced, thereby limiting the adhesion of bacteria to the pyrite or sulfur matrix. Physical friction caused by small particles has the potential to destroy the structure of the cell, thereby causing its leaching process to be inactivated by oxidation. The complex mineralogy of laterite nickel ore also enhances ionic and hydrogen bonding. These effects are affected by small particle size particles where large surface areas expose each particle to a large number of different minerals. Therefore, think -38
m is the critical minimum particle size in this study. However, the actual effective particle size for the nickel recovery should also be determined in subsequent optimization experiments.

Figure 5 Effect of particle size on nickel recovery (combined from basic design and folding design)
3, pulp concentration
The effect of slurry concentration is shown in Figure 6. As can be seen from the figure, the recovery of nickel is high at a low pulp concentration of 5%. The decrease in the leaching rate at high pulp concentrations is due to the uneven mixing of solids and liquids, resulting in gas transport limitations, which are not efficiently transported to the cells due to the liquid being too concentrated (high viscosity).

Figure 6 Effect of slurry concentration on nickel recovery (combined from basic design and folding design)
4, the impact of matrix type
The effect of matrix type is shown in Figure 7. The figure shows that the recovery of nickel is higher when sulfur is used as the substrate. This can be seen in the variation of the use of sulfur ratio with pyrite (Figure 8). Figure 8 is a graph showing the change in pH from the set point during bioleaching using sulfur and pyrite as the matrix in different tests in the basic design. Sulfur was used as the substrate at the time, regardless of the initial pH (1.5 or 2.5), the pH was lowered, and then maintained at a defined level. For a given value of higher pH, the limit value falls within the range of 1.9 to 2.0, and when the given value is lower, the limit value falls within the range of 1.3 to 1.4. When pyrite is used as the matrix, the pH is increased, and when the given value is a lower pH, the pH falls within the range of 1.5 to 1.6. However, for a given value of higher pH, the pH falls within the range of 2.3 to 2.4.

Figure 7 Effect of matrix type on nickel recovery (combined from basic design and folding design)

Figure 8 pH change trend chart (basic design)
1-pH baseline; 2- with pyrite, pH 1.5; 3- with sulfur, pH 1.5;
4-sulfur, pH 2.5; 5--with pyrite, pH 2.5
A similar trend of pH change was observed during the folding design (no results shown). When sulfur is used as the matrix, the limit value falls within the range of 1.3 to 1.4 for a given value of lower pH, and the limit value falls within the range of 1.9 to 2.0 for a given value of higher pH. When pyrite is used as the matrix, the pH falls within the range of 2.1 to 2.2 for a given value and the pH falls within the range of 1.5 to 1.6 for a given value.
In summary, the test results show that the addition of elemental sulfur and subsequent bio-acid production processes are important for maintaining the low pH of laterite nickel ore bioleaching.
5, the impact of bacterial inoculum
The amount of bacterial inoculum seems to have little effect on the leaching process (Figure 1). This is because bacteria do not directly etch the mineral itself. Unlike sulfide ore, the sulfur and ferrous iron that make up the mineral are oxidized, thus having a direct impact on mineral dissolution. The effect of bacteria on mineral dissolution appears to be related to the bacteria and the matrix added to the medium. As can be seen from the study, regardless of the amount of bacterial inoculum, the rate of acid production by bacterial oxidizing sulfur is greater than the rate of acid consumption.
6. Influence of factor interaction
Within the range studied, the interactions between the variables were statistically insignificant except for the slurry concentration and matrix type. Their effects are zero-mean normal distribution, and a straight line appears when plotting (Figure 1). However, the reason for the interaction between the slurry concentration and the matrix type cannot be seen here.
(three) leaching curve
The results of eight (Table 3) laterite nickel ore bioleaching tests are shown in Fig. 9. As can be seen from the figure, a large amount of discrete data was observed in the experiment. This indicates that there is still a large deviation between the factors considered in this study. In some cases, the reduction in recovery is due to the precipitation of nickel ions. A similar leaching curve was observed in the folded design (this result is not shown).

Figure 9 Nickel recovery rate as a function of leaching time (basic design)
Third, conclusions and future research
The purpose of this two-level design is to obtain experimental data on the bioleaching of laterite nickel ore with chemically-inorganic autotrophic microorganisms, optimize them, and determine which factors are significant for response, and which factors are positive influencing factors and which It is a negative factor. From the test results, pH, particle size, slurry concentration and matrix type are statistically important operational parameters. When the bacterial inoculum is between 15% and 5% v/v, it is statistically insignificant. In the range studied, the statistical effect of the exchange between variables was insignificant except for the concentration of the slurry and the type of matrix.
The folding test clarified the main impact results, ensuring that the main factors are not confused with the statistically unimportant two-factor interaction (Figure 1).
The test results show that the results obtained with sulfur as a matrix are better than those obtained with pyrite as a matrix. The pH of the slurry obtained from the sulfur matrix is ​​lower than that when using pyrite, and the production of acid will enhance the recovery of nickel. It is worth noting that the next study will focus on the amount of sulfur added to the leaching medium in order to optimize nickel recovery. The results also show that nickel recovery is highest at low pH and slurry concentrations. Within the range studied, the particle size is less than 38
The particles of m have a negative impact on the recovery of nickel.
The test results show that there may be curvature in the influence curve. Therefore, not only the linear impact assessment but also the quadratic impact assessment should be performed to establish the response surface of the region with the highest nickel recovery rate defined by the diagnostic test. Conduct a new three-level test design.
Cooling Pipe
Cooling pipe, a new solution for heat transfer. It's widely used in different ranges of heat exchanger or collection, such as new energy automobile / vehicles ( cylindrical battery, square battery, soft packing battery), solar panel, solar energy collector, photovoltaic power generation collector, soaking plate, temperature uniforming plate....
It`s made by extruded tube, the joining method is brazing, welding and other suitable techniques.
Features:
Extremely high performance and thermal uniformity
Aluminum alloy material, lightweight
Fast heat dissipation
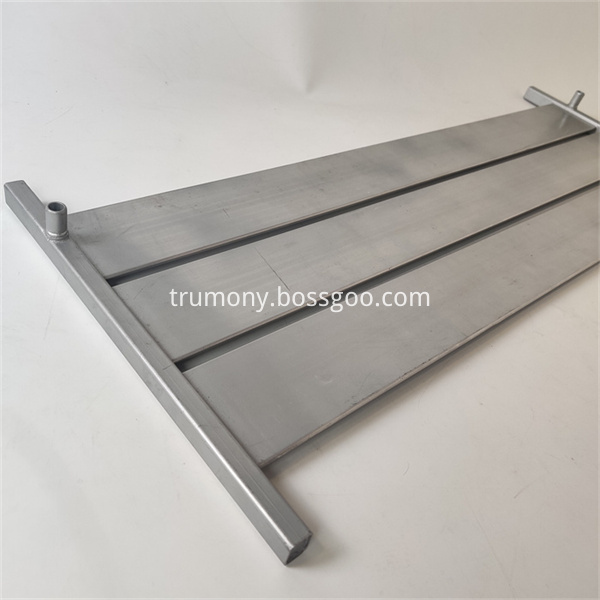
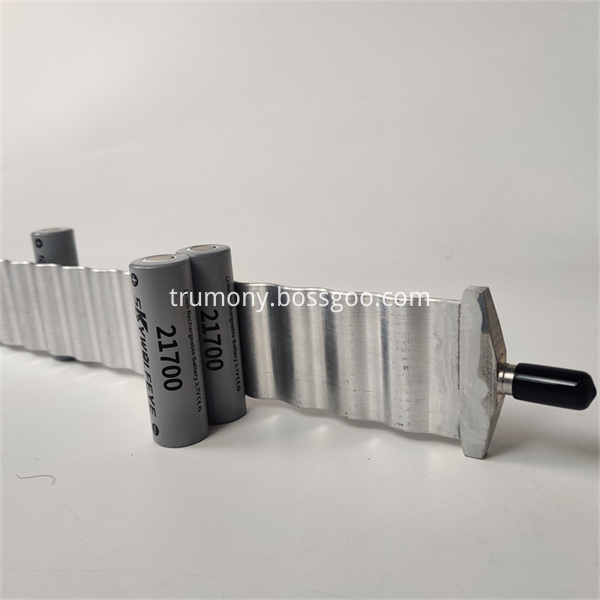
inverter cooling solution,heat pipe water cooling,water pipe cooler
Trumony Aluminum Limited , https://www.szaluminumplate.com